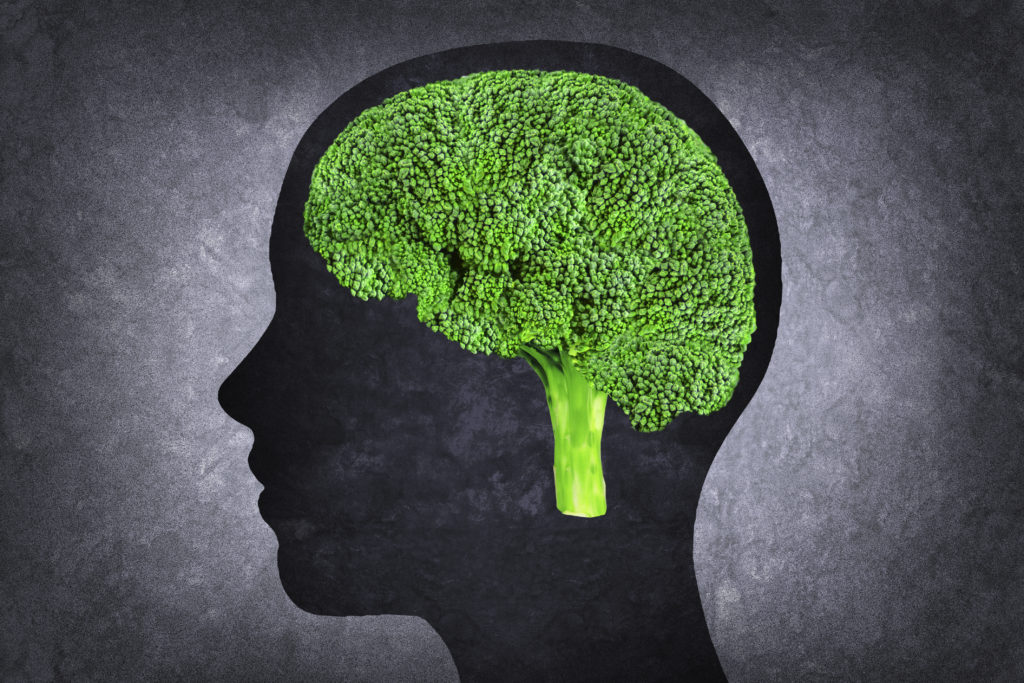
3 minute read
The human brain constitutes about two percent of body mass yet consumes approximately 20% of available blood sugar (Mergenthaler et al., 2013). Unlike other major energy consumers such as muscle and liver cells, neurons store very little glucose, certainly not enough to avoid a shortage (Dienel & Rothman, 2019). In addition, while both muscle and liver cells can utilize fat as an energy reserve, neurons cannot (Newsholme & Leech, 2010). When energy demands for the body are high, for example during rigorous exercise, utilization of glucose increases in the brain as well. Since neurons cannot survive without energy, the question becomes: What’s the backup plan?
It has only been within the past decade or so that new imaging technologies have enabled scientists to propose answers to this question. They have developed three theories: not necessarily exclusive of each other. The first is that the brain can utilize ketone bodies (Newsholme & Leech, 2010), particularly during periods of hypoglycemia or starvation. Neurologists have also discovered that unlike neurons, astrocytes- glial cells that outnumber neurons in the brain- cannot only store glycogen, they can also produce it (Sofroniew & Vinters, 2010; Dienel & Rothman, 2019).
Although glucose crosses the blood brain barrier, quantities are not large enough to support metabolic function when energy demands are high (Brown et al., 2019). The job of keeping neurons “fed” is largely accomplished by astrocytes via one of two mechanisms. One is that by producing and utilizing glycogen for their own demands, astrocytes spare glucose for neurons (Dienel & Rothman, 2019). The second theory is the so-called astrocyte-neuron-lactate shuttle (ANLS), in which astrocytes convert stored glycogen to lactate, which neurons that utilize as fuel (Mathieu et al., 2019).
Why the conversion of glycogen to lactate? Glycogen molecules are large-branched structures; too large to shuttle through the intercellular spaces between astrocytes and neurons, hence the need for a smaller molecule (Mathieu et al., 2019). Although scientists have varying opinions about these theories, all agree on one important point: this backup energy system is a brilliant evolutionary solution to a difficult problem: the need to supply large amounts of energy to a vital organ with limited storage space. That indeed is food for thought.
References
Brown, A., Rich, L. & Ransom, B. (2019). Metabolism of glycogen in brain white matter. In DiNuzzo & Schousboe, (Eds.). Brain Glycogen Metabolism, (pp. 187-208), Springer Nature. http://www.springer.com
Dienel, G. & Rothman, D. (2019). Glycogenolysis in cerebral cortex during sensory stimulation, acute hypoglycemia and exercise: impact on astrocyte-neuron interactions. In DiNuzzo & Schousboe, (Eds.). Brain Glycogen Metabolism, (pp. 209-268), Springer Nature. http://www.springer.com
Mathieu, C., Dupret, J. & Rodrigues-Lima, F. (2019). The structure and regulation of glycogen phosphorylases in brain. In DiNuzzo & Schousboe, (Eds.). Brain Glycogen Metabolism, (pp. 125-147), Springer Nature. http://www.springer.com
Mergenthaler, P. et al. (2013). Sugar for the brain: the role of glucose in physiological and pathological brain function. Trends Neurosci, 36 (10), 587-597. https://doi.org/ 10.1016/j.tins.2013.07.001
Newsholme, E. & Leech, T. (2010). Functional Biochemistry in Health and Disease. Wiley Blackwell. http://www.wiley.com/wiley-blackwell
Sofroniew, M. & Vinters, H. (2009). Astrocytes: biology and pathology. Acta Neuropathologica, 119 (1), 7-35. https://www.doi.org/ 10.1007/s00401-009-0619-8
Leave a Reply